Air Quality Impacts
Key Findings
Key Finding 1: Exacerbated Ozone Health Impacts
Key Finding 2: Increased Health Impacts from Wildfires
Wildfires emit fine particles and
Key Finding 3: Worsened Allergy and Asthma Conditions
Changes in
Changes in the
Poor air quality, whether outdoors or indoors, can negatively affect the human
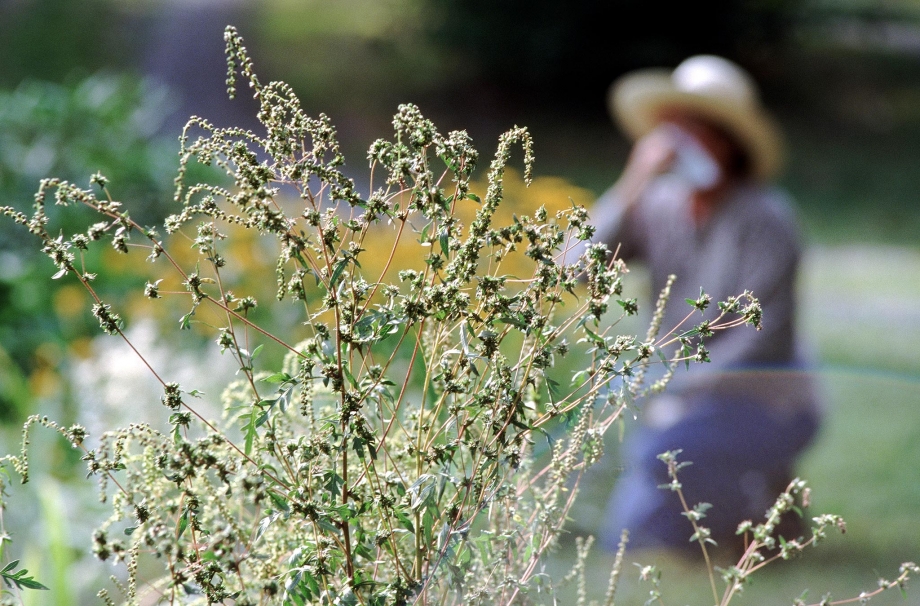
Higher pollen concentrations and longer pollen seasons can
increase allergic sensitization and
© Roy Morsch/CORBIS
A changing climate can also influence the level of aeroallergens such as pollen, which in turn adversely affect human health. Rising levels of CO2 and resulting climate changes alter the production, allergenicity (a measure of how much particular allergens, such as ragweed, affect people), distribution, and seasonal timing of aeroallergens. These changes increase the severity and
Finally, climate change may alter the indoor concentrations of pollutants generated outdoors (such as ground-level ozone), particulate matter, and aeroallergens (such as pollen). Changes in the climate may also increase pollutants generated indoors, such as mold and volatile organic compounds. Most of the air people breathe over their lifetimes will be indoors, since people spend the vast majority of their time in indoor environments. Thus, alterations in indoor air pollutant concentrations from climate change have important health implications.
Figure 3.1: Climate Change and Health—Outdoor Air Quality
Changes in the
Ground-Level Ozone
Ozone levels and subsequent ozone-related
Meteorological conditions influencing ozone levels include air temperatures, humidity, cloud cover, precipitation, wind trajectories, and the amount of vertical mixing in the atmosphere.1,2,25,26 Higher temperatures can increase the chemical rates at which ozone is formed and increase ozone precursor emissions from anthropogenic sources and biogenic(vegetative)sources. Lower relative humidity reduces cloud cover and rainfall, promoting the formation of ozone and extending ozone lifetime in the atmosphere. A changing climate will also modify wind patterns across the United States, which will influence local ozone levels. Over much of the country, the worst ozone episodes tend to occur when the local air mass does not change over a period of several days, allowing ozone and ozone precursor emissions to accumulate over time.27,28 Climate change is already increasing the frequency of these types of stagnation events over parts of the United States,3 and further increases are projected.29 Ozone concentrations near the ground are strongly influenced by upward and downward movement of air (“vertical mixing”). For example, high concentrations of ozone near the ground often occur in urban areas when there is downward movement of air associated with high pressure (“subsidence”), reducing the extent to which locally emitted pollutants are diluted in the atmosphere.30 In addition, high concentrations of ozone can occur in some rural areas resulting from downward transport of ozone from the stratosphere or upper troposphere to the ground.31
Aside from the direct meteorological influences, there are also indirect impacts on U.S. ozone levels from other climate-influenced factors. For instance, higher water vapor concentrations due to increased temperatures will increase the natural rate of ozone depletion, particularly in remote areas,32 thus decreasing the
There is natural year-to-year variability in temperature and other meteorological factors that influence ozone levels.7 While global average temperature over 30-year climatic timescales is expected to increase, natural interannual variability will continue to play a significant role in year-to-year changes in temperature.35 Over the next several decades, the influence of climate change on meteorological parameters affecting average levels of ozone is expected to be smaller than the natural interannual variability.36
To address these issues, most assessments of climate impacts on meteorology and associated ozone formation concurrently simulate global and regional chemical transport over multiple years using “coupled” models. This approach can isolate the influence of meteorology in forming ozone from the effect of changes in emissions. The consensus of these model-based assessments is that accelerated rates of photochemical reaction, increased occurrence of stagnation events, and other direct meteorological influences are likely to lead to higher levels of ozone over large portions of the United States.8,14,16,17 At the same time, ozone levels in certain regions are projected to decrease as a result of climate change, likely due to localized increases in cloud cover, precipitation, and/or increased dilution resulting from deeper mixed layers. These climate-driven changes in projected ozone vary by season and location, with climate and air quality models showing the most consistency in ozone increases due to climate change in the northeastern United States.8,37
Generally, ozone levels will likely increase across the United States if ozone precursors are unchanged (see “Research Highlight: Ozone-Related Health Effects”) .4,7,8 This climate penalty for ozone will offset some of the expected health benefits that would otherwise result from the expected ongoing reductions of ozone precursor emissions, and could prompt the need for adaptive measures (for example, additional ozone precursor emissions reductions) to meet national air quality goals.
Air pollution
Air pollution health impact assessments combine risk estimates from these epidemiology studies with modeled changes in future or historical air quality changes to estimate the number of air-pollution-related premature deaths and illness.41 Future ozone-related human health impacts attributable to climate change are projected to lead to hundreds to thousands of premature deaths, hospital admissions, and cases of acute respiratory illnesses per year in the United States in 2030.14,42,43,44,45,46
Health outcomes that can be attributed to climate change impacts on air pollution are sensitive to a number of factors noted above—including the climate models used to describe meteorological changes (including precipitation and cloud cover), the models simulating air quality levels (including
Particulate Matter
Particulate matter (PM) is a complex mixture of solid- or liquid-phase substances in the atmosphere that arise from both natural and human sources. Principal constituents of PM include sulfate, nitrate, ammonium, organic carbon, elemental carbon, sea salt, and dust. These particles (also known as aerosols) can either be directly emitted or can be formed in the atmosphere from gas-phase precursors. PM smaller than 2.5 microns in diameter (PM2.5) is associated with serious chronic and acute health effects, including lung cancer,
As is the case for ozone, atmospheric PM2.5 concentrations depend on emissions and on meteorology. Emissions of sulfur dioxide (
Climate change is expected to alter several meteorological factors that affect PM2.5, including precipitation patterns and humidity, although there is greater consensus regarding the effects of meteorological changes on ozone than on PM2.5.2 Several factors, such as increased humidity, increased stagnation events, and increased biogenic emissions are likely to increase PM2.5 levels, while increases in precipitation, enhanced atmospheric mixing, and other factors could decrease PM2.5 levels.2,8,37,62 Because of the strong influence of changes in precipitation and atmospheric mixing on PM2.5 levels, and because there is more variability in projected changes to those variables, there is no consensus yet on whether meteorological changes will lead to a net increase or decrease in PM2.5 levels in the United States.2,8,17,21,22,62,63
As a result, while it is clear that PM2.5 accounts for most of the health burden of outdoor air pollution in the United States,10 the health effects of climate-induced changes in PM2.5 are poorly quantified. Some studies have found that changes in PM2.5 will be the dominant driver of air quality-related health effects due to climate change,44 while others have suggested a potentially more significant health burden from changes in ozone.50
PM resulting from natural sources (such as plants, wildfires, and dust) is sensitive to daily weather patterns, and those fluctuations can affect the intensity of extreme PM episodes (see also Ch. 4: Extreme Events, Section 4.6).8 Wildfires are a major source of PM, especially in the western United States during summer.64,65,66 Because winds carry PM2.5 and ozone precursor gases, air pollution from wildfires can affect people even far downwind from the fire location.35,67 PM2.5 from wildfires affects human health by increasing the risk of premature death and hospital and emergency department visits.68,69,70
Climate change has already led to an increased frequency of large wildfires, as well as longer durations of individual wildfires and longer wildfire seasons in the western United States.71 Future climate change is projected to increase wildfire risks72,73 and associated emissions, with harmful impacts on health.74 The area burned by wildfires in North America is expected to increase dramatically over the 21st century due to climate change.75,76 By 2050, changes in wildfires in the western United States are projected to result in 40% increases of organic carbon and 20% increases in elemental carbon aerosol concentrations.77 Wildfires may dominate summertime PM2.5 concentrations, offsetting even large reductions in anthropogenic PM2.5 emissions.22
Likewise, dust can be an important constituent of PM, especially in the southwest United States. The severity and spatial extent of
Aeroallergens and Rates of Allergic Diseases in the United States
Aeroallergens are substances present in the air that, once inhaled, stimulate an allergic response in sensitized individuals. Aeroallergens include tree, grass, and weed pollen; indoor and outdoor molds; and other allergenic proteins associated with animal dander, dust mites, and cockroaches.83 Ragweed is the aeroallergen that most commonly affects persons in the United States.84
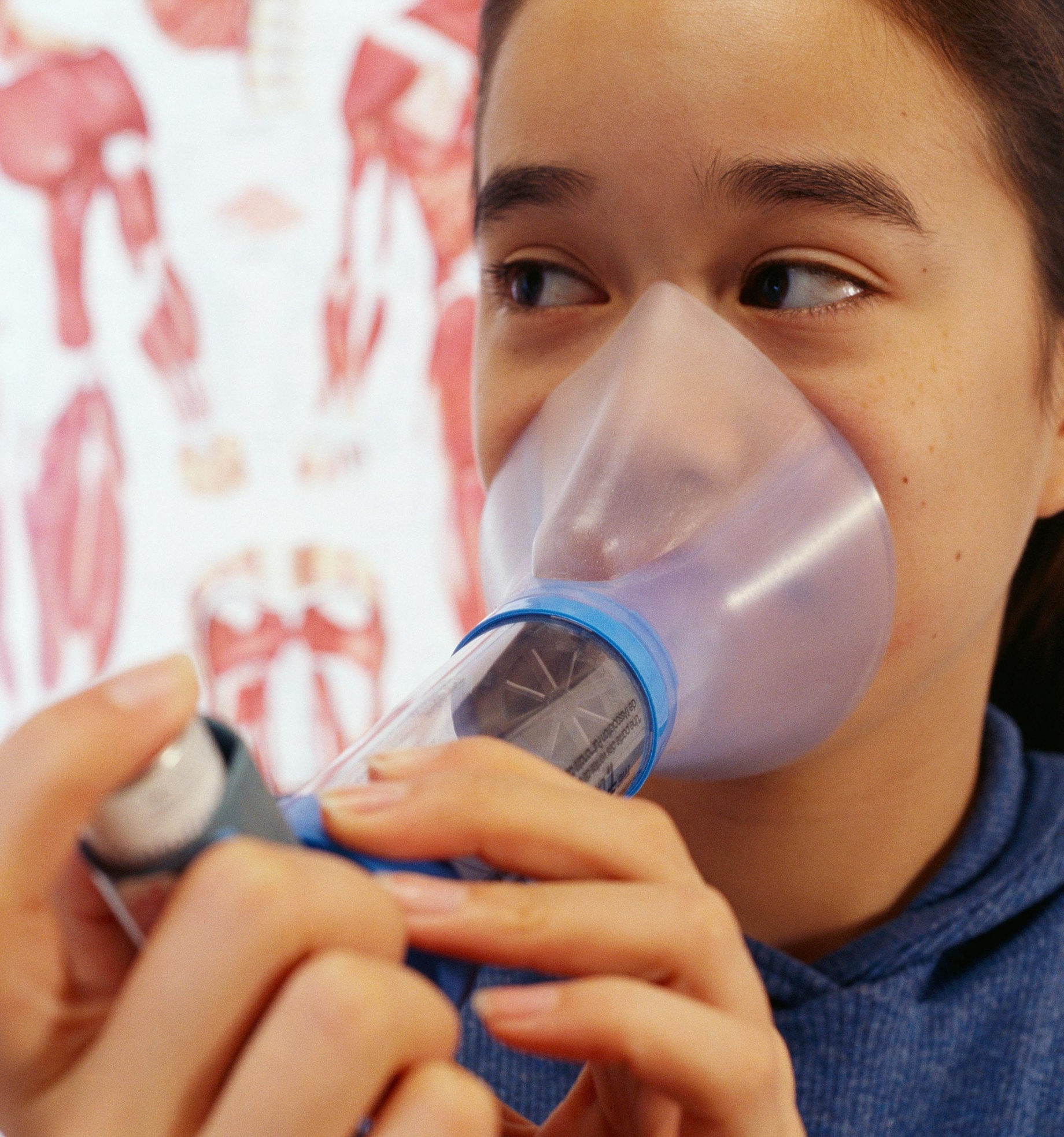
Nearly 6.8 million children in the United States are affected by
© Stephen Welstead/LWA/Corbis
Allergic diseases develop in response to complex and multiple interactions among both genetic and non-genetic factors, including a developing immune system, environmental exposures (such as ambient air pollution or
Climate Impacts on Aeroallergen Characteristics
Climate change contributes to changes in allergic illnesses as greater concentrations of
Historical trends show that climate change has led to changes in the length of the growing season for certain allergenic pollens. For instance, the duration of pollen release for common ragweed (Ambrosia artemisiifolia) has been increasing as a function of latitude in recent decades in the midwestern region of North America (see Figure 3.4). Latitudinal effects on increasing season length were associated primarily with a delay in first frost during the fall season and lengthening of the frost-free period.95 Studies in controlled indoor environments find that increases in temperature and CO2 result in earlier flowering, greater floral numbers, greater pollen production, and increased allergenicity in common ragweed.96,97 In addition, studies using urban areas as proxies for both higher CO2 and higher temperatures demonstrate earlier flowering of pollen species, which may lead to a longer total pollen season.98,99,100
Figure 3.4: Ragweed Pollen Season Lengthens
For trees, earlier flowering associated with higher winter and spring temperatures has been observed over a 50-year period for oak.101 Research on loblolly pine (Pinus taeda) also demonstrates that elevated CO2 could induce earlier and greater seasonal pollen production.102 Annual birch (Betula) pollen production and peak values from 2020 to 2100 are projected to be 1.3 to 2.3 times higher, relative to average values for 2000, with the start and peak dates of pollen release advancing by two to four weeks.103
Climate Variability and Effects on Allergic Diseases
Climate change related alterations in local weather patterns, including changes in minimum and maximum temperatures and rainfall, affect the burden of allergic diseases.104,105,106 The role of weather on the initiation or exacerbation of allergic symptoms in sensitive persons is not well understood.86,107 So-called “thunderstorm asthma” results as allergenic particles are dispersed through osmotic rupture, a phenomenon where cell membranes burst. Pollen grains may, after contact with rain, release part of their cellular contents, including allergen-laced fine particles. Increases in the intensity and frequency of heavy rainfall and storminess over the coming decades is likely to be associated with spikes in aeroallergen concentrations and the potential for related increases in the number and severity of allergic illnesses.108,109
Potential non-linear interactions between aeroallergens and ambient air pollutants (including
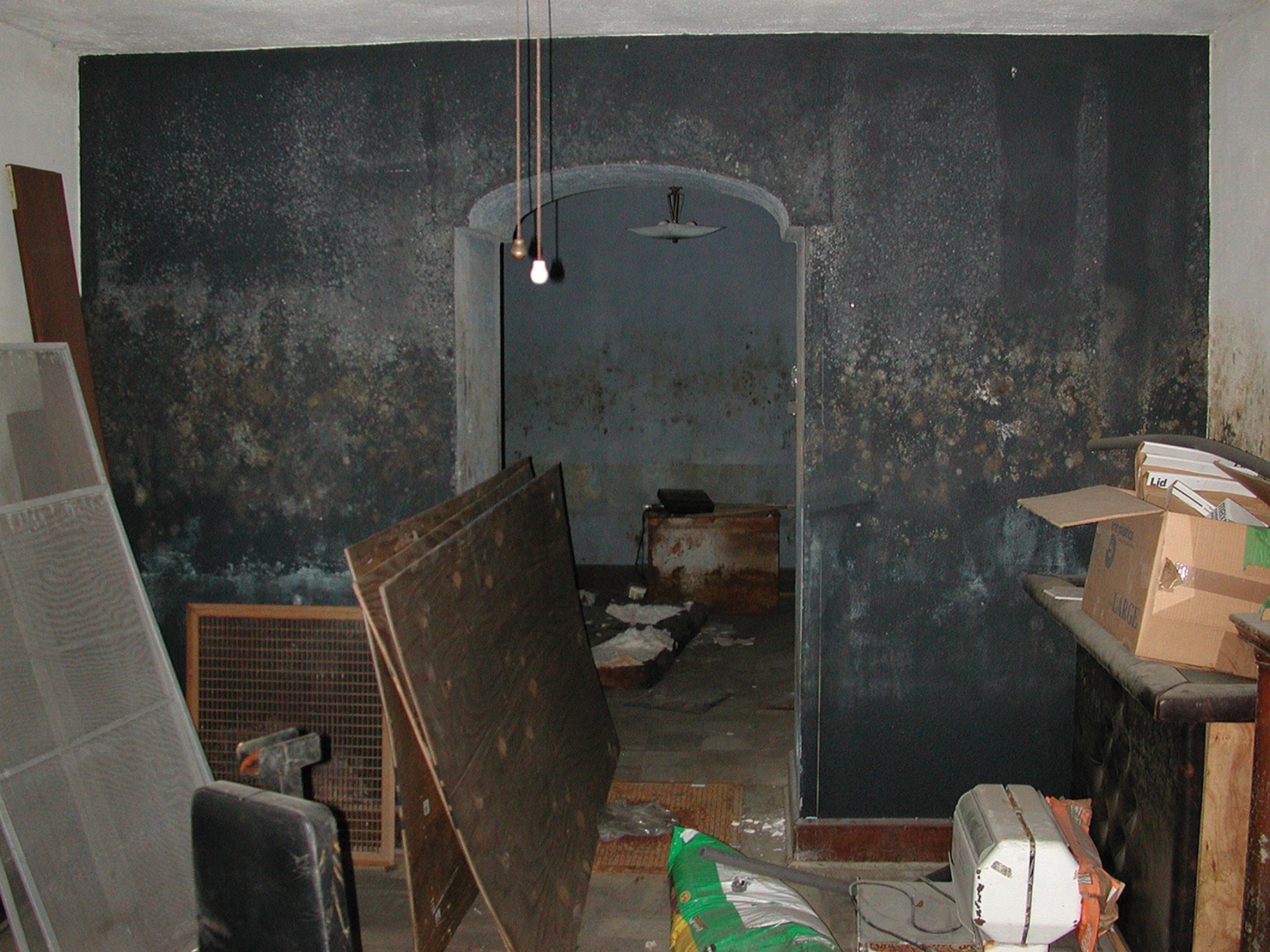
Dampness and mold in U.S. homes are linked to approximately 4.6 million cases of worsened
© Bart Everson/ flickr
Indoor air contains a complex mixture of chemical and biological pollutants or contaminants. Contaminants that can be found indoors include
Public and environmental
Outdoor Air Changes Reflected in Indoor Air
Indoor air pollutants may come from indoor sources or may be transported into the building with outdoor air.127,128 Indoor pollutants of outdoor origin may include
Most exposures to high levels of ozone occur outdoors; however, indoor exposures, while lower, occur for much longer time periods. Indoors, ozone concentrations are usually about 10% to 50% of outdoor concentrations; however, since people spend most of their time indoors, most of their exposure to ozone is from indoor air.130 Thus, about 45% to 75% of a person’s overall exposure to ozone will occur indoors.132 About half of the health effects resulting from any outdoor increases in ozone (see “Aeroallergens and Rates of Allergic Diseases in the United States”) will be due to indoor ozone exposures.130 The elderly and children are particularly sensitive to short-term ozone exposure; however, they may spend even more time indoors than the general population and consequently their exposure to ozone is at lower levels for longer periods than the general public.133,134 In addition, ozone entering a building reacts with some organic compounds to produce secondary indoor air pollutants. These reactions lower indoor ozone concentrations but introduce new indoor air contaminants, including other respiratory irritants.135
Climate-related increases in droughts and dust storms may result in increases in indoor transmission of dust-borne pathogens, as the dust penetrates the indoor environment. Dust contains particles of biologic origin, including pollen and bacterial and fungal spores. Some of the particles are allergenic.136 Pathogenic fungi and
Legionnaires’ disease is primarily contracted from aerosolized water contaminated with Legionella bacteria.139 Legionella bacteria are naturally found outdoors in water and soil; they are also known to contaminate treated water systems in buildings,140 as well as building cooling systems such as swamp coolers or cooling towers.141 Legionella can also be found indoors inside plumbing fixtures such as showerheads, faucets, and humidifiers.142,143 Legionella can cause outbreaks of a pneumonia known as Legionnaire’s disease, which is a potentially fatal infection.144 Exposure can occur indoors when a spray or mist of contaminated water is inhaled, including mist or spray from showers and swamp coolers.145 The spread of Legionella bacteria can be affected by regional environmental factors.116 Legionnaires’ disease is known to follow a seasonal pattern, with more cases in late summer and autumn, potentially due to warmer and damper conditions.146,147 Cases of Legionnaires’ disease are rising in the United States, with an increase of 192% from 2000 to 2009.148,149 If climate change results in sustained higher temperatures and damper conditions in some areas, there could be increases in the spread and transmission of Legionella.
Contaminants Generated Indoors
Although research directly linking indoor dampness and climate change is not available, information on building science, climate change, and outdoor environmental factors that affect indoor air quality can be used to project how climate change may influence indoor environments.130 Climate change could result in increased indoor dampness in at least two ways: 1) if there are more frequent heavy precipitation events and other severe weather events (including high winds, flooding, and winter storms) that result in damage to buildings, allowing water or moisture entry; and 2) if outdoor humidity rises with climate change, indoor humidity and the potential for condensation and dampness will likely rise. Outdoor humidity is usually the largest contributor to indoor dampness on a yearly basis.127 Increased indoor dampness and humidity will in turn increase indoor mold, dust mites, bacteria, and other bio-contamination indoors, as well as increase levels of volatile organic compounds (VOCs) and other chemicals resulting from the off-gassing of damp or wet building materials.116,119,150 Dampness and mold in U.S. homes are linked to approximately 4.6 million cases of worsened asthma and between 8% and 20% of several common respiratory infections, such as
Additionally, power outages due to more frequent extreme weather events such as flooding could lead to a number of health effects (see Ch. 4: Extreme Events). Heating, ventilation, and air conditioning (HVAC) systems will not function without power; therefore, many buildings could have difficulty maintaining indoor temperatures or humidity. Loss of ventilation, filtration, air circulation, and humidity control can lead to indoor mold growth and increased levels of indoor contaminants,153 including VOCs such as formaldehyde.119,154,155,156 Power outages are also associated with increases in hospital visits from carbon monoxide (CO) poisoning, primarily due to the incorrect use of backup and portable generators that contaminate indoor air with carbon monoxide.135 Following floods, CO poisoning is also associated with the improper indoor use of wood-burning appliances, and other combustion appliances designed for use outdoors.157 There were at least nine deaths from carbon monoxide poisoning related to power outages from 2000 to 2009.158
Climate factors can influence populations of rodents that produce allergens and can harbor pathogens such as hantaviruses, which can cause Hantavirus Pulmonary Syndrome. Hantaviruses can be spread to people by rodents that infest buildings,159 and limiting indoor exposure is a key strategy to prevent the spread of hantavirus.160 Climate change may increase rodent populations in some areas, including indoors, particularly when droughts are followed by periods of heavy rain (see Ch. 4: Extreme Events) and with increases in temperature and rainfall.161 Also, extreme weather events such as heavy rains and flooding may drive some rodents to relocate indoors.162 Increases in rodent populations may result in increased indoor exposures to rodent allergens and related health effects.159,163,164 In addition, climate factors may also influence the
Certain groups of people may be more susceptible to harm from air pollution due to factors including age, access to healthcare,
Nearly 6.8 million children in the United States are affected by
Table 3.1: Percentage of population with active asthma, by year and selected characteristics: United States, 2001 and 2010.
Characteristic | Year 2001 % | Year 2010 % |
---|---|---|
Total | 7.3 | 8.4 |
Gender | ||
Male | 6.3 | 7.0 |
Female | 8.3 | 9.8 |
Race | ||
White | 7.2 | 7.8 |
Black | 8.4 | 11.9 |
Other | 7.2 | 8.1 |
Ethnicity | ||
Hispanic | 5.8 | 7.2 |
Non-Hispanic | 7.6 | 8.7 |
Age | ||
Children (0–17) | 8.7 | 9.3 |
Adults (18 and older) | 6.9 | 8.2 |
Age Group | ||
0–4 years | 5.7 | 6.0 |
5–14 years | 9.9 | 10.7 |
15–34 years | 8.0 | 8.6 |
35–64 years | 6.7 | 8.1 |
65 years and older | 6.0 | 8.1 |
Region | ||
Northeast | 8.3 | 8.8 |
Midwest | 7.5 | 8.6 |
South | 7.1 | 8.3 |
West | 6.7 | 8.3 |
Federal Poverty Threshold | ||
Below 100% | 9.9 | 11.2 |
100% to < 250% | 7.7 | 8.7 |
250% to < 450% | 6.8 | 8.2 |
450% or higher | 6.6 | 7.1 |
Source: Moorman et al. 2012179
A recent study of children in California found that racial and ethnic minorities are more affected by asthma.174 Among minority children, the
Minority adults and children also bear a disproportionate burden associated with asthma as measured by emergency department visits, lost work and school days, and overall poorer health status (see Table 3.1).174,175 Twice as many Black children had asthma-related emergency department visits and hospitalizations compared with White children. Fewer Black and Hispanic children reported using preventative medication like inhaled corticosteroids (
Children living in poverty were 1.75 times more likely to be hospitalized for asthma than their non-poor counterparts. When income is accounted for, no significant difference was observed in the rate of hospital admissions by race or ethnicity. This income effect may be related to access and use of health care and appropriate use of preventive medications such as ICS.177
People with preexisting medical conditions—including
In addition to the emerging issues identified above, the authors highlight the following potential areas for additional scientific and research activity on air quality. Understanding of future air quality and the ability to model future
Future assessments can benefit from research activities that:
- enhance understanding of how interactions among climate-related factors, such as temperature or relative humidity,
aeroallergens , and air pollution, affect human health, and how to attribute health impacts to changes in these differentrisk factors; - improve the ability to model and project climate change impacts on the formation and fate of air contaminants and quantify the compounded
uncertainty in the projections; and - identify the impacts of changes in indoor dampness, such as mold, other biological contaminants, volatile organic compounds, and indoor air chemistry on indoor air pollutants and health.
References
- Allergy Statistics. American Academy of Allergy, Asthma and Immunology. URL | Detail cited 2013:
- Moving environmental justice indoors: Understanding structural influences on residential exposure patterns in low-income communities. American Journal of Public Health, 101, S238-S245. doi:10.2105/AJPH.2011.300119 | Detail , 2011:
- Trends in Asthma Prevalence, Health Care Use, and Mortality in the United States, 2001–2010. NCHS Data Brief. No. 94, May 2012. Array, 8 pp., National Center for Health Statistics, Hyattsville, MD. URL | Detail , 2012:
- Projected carbon dioxide to increase grass pollen and allergen exposure despite higher ozone levels. PLoS ONE, 9, e111712. doi:10.1371/journal.pone.0111712 | Detail , 2014:
- Intercontinental impacts of ozone pollution on human mortality. Environmental Science & Technology, 43, 6482-6287. doi:10.1021/es900518z | Detail , 2009:
- Role of outdoor aeroallergens in asthma exacerbations: Epidemiological evidence. Thorax, 59, 277-278. doi:10.1136/thx.2003.019133 | Detail , 2004:
- Air pollution and allergens. Journal of Investigational Allergology and Clinical Immunology, 17 Suppl 2, 3-8. URL | Detail , 2007:
- Impacts of climate change on aeroallergens: Past and future. Clinical & Experimental Allergy, 34, 1507-1513. doi:10.1111/j.1365-2222.2004.02061.x | Detail , 2004:
- Is the global rise of asthma an early impact of anthropogenic climate change? Environmental Health Perspectives, 113, 915-919. doi:10.1289/ehp.7724 | Detail , 2005:
- Ozone and short-term mortality in 95 US urban communities, 1987-2000. JAMA: The Journal of the American Medical Association, 292, 2372-2378. doi:10.1001/jama.292.19.2372 | Detail , 2004:
- Effect modification by community characteristics on the short-term effects of ozone exposure and mortality in 98 US communities. American Journal of Epidemiology, 167, 986-997. doi:10.1093/aje/kwm396 | Detail , 2008:
- Climate change, ambient ozone, and health in 50 US cities. Climatic Change, 82, 61-76. doi:10.1007/s10584-006-9166-7 | Detail , 2007:
- Who is more affected by ozone pollution? A systematic review and meta-analysis. American Journal of Epidemiology, 180, 15-28. doi:10.1093/aje/kwu115 | Detail , 2014:
- Determining the infiltration of outdoor particles in the indoor environment using a dynamic model. Journal of Aerosol Science, 37, 766-785. doi:10.1016/j.jaerosci.2005.05.020 | Detail , 2006:
- The potential impacts of climate variability and change on air pollution-related health effects in the United States. Environmental Health Perspectives, 109, 199-209. | Detail , 2001:
- Climate changes, environment and infection: Facts, scenarios and growing awareness from the public health community within Europe. Anaerobe, 17, 337-340. doi:10.1016/j.anaerobe.2011.05.016 | Detail , 2011:
- Climate change and allergic disease. Current Allergy and Asthma Reports, 12, 485-494. doi:10.1007/s11882-012-0314-z | Detail , 2012:
- Anthropogenic climate change and allergic diseases. Atmosphere, 3, 200-212. doi:10.3390/atmos3010200 | Detail , 2012:
- Summary Health Statistics for U.S. Children: National Health Interview Survey, 2012. 73 pp., National Center for Health Statistics, Hyattsville, MD. URL | Detail , 2013:
- Observed relationships of ozone air pollution with temperature and emissions. Geophysical Research Letters, 36, L09803. doi:10.1029/2009gl037308 | Detail , 2009:
- Seasonal Variability in Bacterial and Fungal Diversity of the Near-Surface Atmosphere. Environmental Science & Technology, 47, 12097-12106. doi:10.1021/es402970s | Detail , 2013:
- Unplanned airflows & moisture problems. ASHRAE journal, 44, 44-49. URL | Detail , 2002:
- Relationship between climate, pollen concentrations of Ambrosia and medical consultations for allergic rhinitis in Montreal, 1994–2002. Science of The Total Environment, 370, 39-50. doi:10.1016/j.scitotenv.2006.05.022 | Detail , 2006:
- Asthma prevalence among US children in underrepresented minority populations: American Indian/Alaska Native, Chinese, Filipino, and Asian Indian. Pediatrics, 122, e217-e222. doi:10.1542/peds.2007-3825 | Detail , 2008:
- Carbon monoxide exposures--United States, 2000-2009. Morbidity and Mortality Weekly Report, 60, 1014-1017. PMID: 21814164 | Detail , 2011:
- Does air pollution increase the effect of aeroallergens on hospitalization for asthma? Journal of Allergy and Clinical Immunology, 129, 228-231. doi:10.1016/j.jaci.2011.09.025 | Detail , 2012:
- The effects of meteorology on ozone in urban areas and their use in assessing ozone trends. Atmospheric Environment, 41, 7127-7137. doi:10.1016/j.atmosenv.2007.04.061 | Detail , 2007:
- Projections of the effects of climate change on allergic asthma: The contribution of aerobiology. Allergy, 65, 1073-1081. doi:10.1111/j.1398-9995.2010.02423.x | Detail , 2010:
- A statistical modeling framework for projecting future ambient ozone and its health impact due to climate change. Atmospheric Environment, 89, 290-297. doi:10.1016/j.atmosenv.2014.02.037 | Detail , 2014:
- Impact of climate change on ambient ozone level and mortality in southeastern United States. International Journal of Environmental Research and Public Health, 7, 2866-2880. doi:10.3390/ijerph7072866 | Detail , 2010:
- Racial and ethnic disparities in asthma medication usage and health-care utilization: Data from the National Asthma Survey. Chest, 136, 1063-1071. doi:10.1378/chest.09-0013 | Detail , 2009:
- Legionnaires' disease. The Lancet, 387, 376-385. doi:10.1016/S0140-6736(15)60078-2 | Detail , 2016:
- Increase in pre-seasonal community-acquired Legionnaire's disease due to increased precipitation. Clinical Microbiology and Infection, 21, e45-e46. doi:10.1016/j.cmi.2015.02.015 | Detail , 2015:
- Climate change, air pollution and extreme events leading to increasing prevalence of allergic respiratory diseases. Multidisciplinary Respiratory Medicine, 8, 1-9. doi:10.1186/2049-6958-8-12 | Detail , 2013:
- The role of outdoor air pollution and climatic changes on the rising trends in respiratory allergy. Respiratory Medicine, 95, 606-611. doi:10.1053/rmed.2001.1112 | Detail , 2001:
- Environmental risk factors and allergic bronchial asthma. Clinical & Experimental Allergy, 35, 1113-1124. doi:10.1111/j.1365-2222.2005.02328.x | Detail , 2005:
- Urban air pollution and climate change as environmental risk factors of respiratory allergy: An update. Journal of Investigational Allergology and Clinical Immunology, 20, 95-102. URL | Detail , 2010:
- Environmental urban factors (air pollution and allergens) and the rising trends in allergic respiratory diseases. Allergy, 57, 30-33. doi:10.1034/j.1398-9995.57.s72.5.x | Detail , 2002:
- Effects of climate change on environmental factors in respiratory allergic diseases. Clinical & Experimental Allergy, 38, 1264-1274. doi:10.1111/j.1365-2222.2008.03033.x | Detail , 2008:
- Climate change, migration, and allergic respiratory diseases: An update for the allergist. World Allergy Organization Journal, 4, 121-125. doi:10.1097/WOX.0b013e3182260a57 | Detail , 2011:
- Thunderstorm-asthma and pollen allergy. Allergy, 62, 11-16. doi:10.1111/j.1398-9995.2006.01271.x | Detail , 2007:
- A comparison of CMAQ-based and observation-based statistical models relating ozone to meteorological parameters. Atmospheric Environment, 45, 3481-3487. doi:10.1016/j.atmosenv.2010.12.060 | Detail , 2011:
- Understanding the meteorological drivers of U.S. particulate matter concentrations in a changing climate. Bulletin of the American Meteorological Society, 95, 521-532. doi:10.1175/BAMS-D-12-00181.1 | Detail , 2014:
- Impacts of climate change on regional and urban air quality in the eastern United States: Role of meteorology. Journal of Geophysical Research: Atmospheres, 114. doi:10.1029/2008JD009849 | Detail , 2009:
- Ecology of hantavirus in a changing world. Annals of the New York Academy of Sciences, 1195, 99-112. doi:10.1111/j.1749-6632.2010.05452.x | Detail , 2010:
- Hospital water and opportunities for infection prevention. Current Infectious Disease Reports, 16, 432. doi:10.1007/s11908-014-0432-y | Detail , 2014:
- The relationship of respiratory and cardiovascular hospital admissions to the southern California wildfires of 2003. Occupational and Environmental Medicine, 66, 189-197. doi:10.1136/oem.2008.041376 | Detail , 2009:
- Effects of ambient pollen concentrations on frequency and severity of asthma symptoms among asthmatic children. Epidemiology, 23, 55-63. doi:10.1097/EDE.0b013e31823b66b8 | Detail , 2012:
- Climate change and children. Pediatric Clinics of North America, 54, 213-226. doi:10.1016/j.pcl.2007.01.004 | Detail , 2007:
- Regulatory Impact Analysis - Control of Air Pollution from New Motor Vehicles: Tier 2 Motor Vehicle Emissions Standards and Gasoline Sulfur Control Requirements. 522 pp., U.S. Environmental Protection Agency, Office of Transportation and Air Quality, Washington, D.C. URL | Detail 1999:
- Review of the Impact of Climate Variability and Change on Aeroallergens and Their Associated Effects. EPA/600/R-06/164F. 125 pp., U.S. Environmental Protection Agency, Washington, D.C. URL | Detail 2008:
- Integrated Science Assessment for Particulate Matter. Array, National Center for Environmental Assessment, Office of Research and Development, U.S. Environmental Protection Agency, Research Triangle Park, NC. URL | Detail 2009:
- Integrated Science Assessment for Ozone and Related Photochemical Oxidants. Array, 1251 pp., U.S. Environmental Protection Agency, National Center for Environmental Assessment, Office of Research and Development, Research Triangle Park, NC. URL | Detail 2013:
- Regulatory Impact Analysis of the Proposed Revisions to the National Ambient Air Quality Standards for Ground-Level Ozone. U.S. Environmental Protection Agency, Office of Air and Radiation, Office of Air Quality Planning and Standards, Research Triangle Park, NC. URL | Detail 2014:
- Cross-State Air Pollution Rule (CSAPR). U.S. Environmental Protection Agency. URL | Detail cited 2015:
- Tier 2 Vehicle and Gasoline Sulfur Program. U.S. Environmental Protection Agency, Office of Transportation and Air Quality. URL | Detail cited 2015:
- Technical Support Document (TSD): Preparation of Emissions Inventories for the Version 6.2, 2011 Emissions Modeling Platform. U.S. Environmental Protection Agency, Office of Air and Radiation. URL | Detail 2015:
- Epidemiology and ecology of opportunistic premise plumbing pathogens: Legionella pneumophila, Mycobacterium avium, and Pseudomonas aeruginosa. Environmental Health Perspectives, 123, 749-758. doi:10.1289/ehp.1408692 | Detail , 2015:
- Estimating the national public health burden associated with exposure to ambient PM2.5 and ozone. Risk Analysis, 32, 81-95. doi:10.1111/j.1539-6924.2011.01630.x | Detail , 2012:
- The geographic distribution and economic value of climate change-related ozone health impacts in the United States in 2030. Journal of the Air & Waste Management Association, 65, 570-580. doi:10.1080/10962247.2014.996270 | Detail , 2015:
- Legionnaires' disease incidence and risk factors, New York, New York, USA, 2001-2011. Emerging Infectious Diseases, 20, 1795-1802. doi:10.3201/eid2011.131872 | Detail , 2014:
- Global air quality and climate. Chemical Society Reviews, 41, 6663-6683. doi:10.1039/C2CS35095E | Detail , 2012:
- Air quality and climate connections. Journal of the Air & Waste Management Association, 65, 645-685. doi:10.1080/10962247.2015.1040526 | Detail , 2015:
- Review of some effects of climate change on indoor environmental quality and health and associated no-regrets mitigation measures. Building and Environment, 86, 70-80. doi:10.1016/j.buildenv.2014.12.024 | Detail , 2015:
- Association of residential dampness and mold with respiratory tract infections and bronchitis: A meta-analysis. Environmental Health, 9, Article 72. doi:10.1186/1476-069x-9-72 | Detail , 2010:
- The impact of emission and climate change on ozone in the United States under representative concentration pathways (RCPs). Atmospheric Chemistry and Physics, 13, 9607-9621. doi:10.5194/acp-13-9607-2013 | Detail , 2013:
- U.S. air quality and health benefits from avoided climate change under greenhouse gas mitigation. Environmental Science & Technology, 49, 7580-7588. doi:10.1021/acs.est.5b01324 | Detail , 2015:
- Quercus pollen season dynamics in the Iberian peninsula: Response to meteorological parameters and possible consequences of climate change. Annals of Agricultural and Environmental Medicine, 13, 209-224. URL | Detail , 2006:
- Ch. 20: Southwest. Climate Change Impacts in the United States: The Third National Climate Assessment, , U.S. Global Change Research Program, 462-486. doi:10.7930/J08G8HMN | Detail , 2014:
- Pediatric asthma: A different disease. Proceedings of the American Thoracic Society, 6, 278-282. doi:10.1513/pats.200808-090RM | Detail , 2009:
- Elevated atmospheric CO2 concentration and temperature across an urban–rural transect. Atmospheric Environment, 41, 7654-7665. doi:10.1016/j.atmosenv.2007.08.018 | Detail , 2007:
- The relationship between meteorological variables and sporadic cases of Legionnaires' disease in residents of England and Wales. Epidemiology & Infection, 142, 2352-2359. doi:10.1017/S0950268813003294 | Detail , 2014:
- Relationship between boundary layer heights and growth rates with ground-level ozone in Houston, Texas. Journal of Geophysical Research: Atmospheres, 119, 6230-6245. doi:10.1002/2013JD020473 | Detail , 2014:
- Adverse human health effects associated with molds in the indoor environment. Journal of Occupational and Environmental Medicine, 45, 470-478. doi:10.1097/00043764-200305000-00006 | Detail , 2003:
- Three measures of forest fire smoke exposure and their associations with respiratory and cardiovascular health outcomes in a population-based cohort. Environmental Health Perspectives, 119, 1266-1271. doi:10.1289/ehp.1002288 | Detail , 2011:
- Legionellosis --- United States, 2000-2009. Morbidity and Mortality Weekly Report, 60, 1083-1086. PMID: 21849965 | Detail , 2011:
- Assessment of relative potential for Legionella species or surrogates inhalation exposure from common water uses. Water Research, 56, 203-213. doi:10.1016/j.watres.2014.02.013 | Detail , 2014:
- Response of air stagnation frequency to anthropogenically enhanced radiative forcing. Environmental Research Letters, 7, 044034. doi:10.1088/1748-9326/7/4/044034 | Detail , 2012:
- Methodological considerations in developing local-scale health impact assessments: Balancing national, regional, and local data. Air Quality, Atmosphere & Health, 2, 99-110. doi:10.1007/s11869-009-0037-z | Detail , 2009:
- Effects of climate change on residential infiltration and air pollution exposure. Journal of Exposure Science and Environmental Epidemiology, Published online 27 May 2015. doi:10.1038/jes.2015.38 | Detail , 2015:
- Indoor Allergens: Assessing and Controlling Adverse Health Effects. Institute of Medicine. The National Academies Press, 350 pp. doi:10.17226/2056 | Detail 1993:
- Clearing the Air: Asthma and Indoor Air Exposures. Institute of Medicine. The National Academies Press, 456 pp. URL | Detail 2000:
- Damp Indoor Spaces and Health. Institute of Medicine. The National Academies Press, 370 pp. doi:10.17226/11011 | Detail 2004:
- Climate Change, the Indoor Environment, and Health. The National Academies Press, 286 pp. doi:10.17226/13115 | Detail 2011:
- Climate Change 2013: The Physical Science Basis. Contribution of Working Group I to the Fifth Assessment Report of the Intergovernmental Panel on Climate Change. 1535 pp., Cambridge University Press, Cambridge, UK and New York, NY. doi:10.1017/CBO9781107415324 | Detail 2013:
- Public health impacts of climate change in Washington State: Projected mortality risks due to heat events and air pollution. Climatic Change, 102, 159-186. doi:10.1007/s10584-010-9852-3 | Detail , 2010:
- Effect of climate change on air quality. Atmospheric Environment, 43, 51-63. doi:10.1016/j.atmosenv.2008.09.051 | Detail , 2009:
- On the causal link between carbon dioxide and air pollution mortality. Geophysical Research Letters, 35, L03809. doi:10.1029/2007GL031101 | Detail , 2008:
- Long-term ozone exposure and mortality. New England Journal of Medicine, 360, 1085-1095. doi:10.1056/NEJMoa0803894 | Detail , 2009:
- Effect modification of ozone-related mortality risks by temperature in 97 US cities. Environment International, 73, 128-134. doi:10.1016/j.envint.2014.07.009 | Detail , 2014:
- Review of health hazards and prevention measures for response and recovery workers and volunteers after natural disasters, flooding, and water damage: Mold and dampness. Environmental Health and Preventive Medicine, 19, 93-99. doi:10.1007/s12199-013-0368-0 | Detail , 2014:
- A global perspective on hantavirus ecology, epidemiology, and disease. Clinical Microbiology Reviews, 23, 412-441. doi:10.1128/cmr.00062-09 | Detail , 2010:
- Health effects of the 2003 southern California wildfires on children. American Journal of Respiratory and Critical Care Medicine, 174, 1221-1228. doi:10.1164/rccm.200604-519OC | Detail , 2006:
- Projections of mid-century summer air-quality for North America: Effects of changes in climate and precursor emissions. Atmospheric Chemistry and Physics, 12, 5367-5390. doi:10.5194/acp-12-5367-2012 | Detail , 2012:
- Fire in the air: Biomass burning impacts in a changing climate. Critical Reviews in Environmental Science and Technology, 43, 40-83. doi:10.1080/10643389.2011.604248 | Detail , 2013:
- Climate change, air quality, and human health. American Journal of Preventive Medicine, 35, 459-467. doi:10.1016/j.amepre.2008.08.025 | Detail , 2008:
- Near-term climate change: Projections and predictability. Climate Change 2013: The Physical Science Basis. Contribution of Working Group I to the Fifth Assessment Report of the Intergovernmental Panel on Climate Change, , Cambridge University Press, 953–1028. doi:10.1017/CBO9781107415324.023 | Detail , 2013:
- Socioeconomic predictors of high allergen levels in homes in the greater Boston area. Environmental Health Perspectives, 108, 301-307. URL | Detail , 2000:
- Emergence and persistence of hantaviruses. Wildlife and Emerging Zoonotic Diseases: The Biology, Circumstances and Consequences of Cross-Species Transmission, , Springer-Verlag, 217-252. doi:10.1007/978-3-540-70962-6_10 | Detail , 2007:
- The National Human Activity Pattern Survey (NHAPS): A resource for assessing exposure to environmental pollutants. Journal of Exposure Analysis and Environmental Epidemiology, 11, 231-252. doi:10.1038/sj.jea.7500165 | Detail , 2001:
- Elevated CO2 and tree fecundity: The role of tree size, interannual variability, and population heterogeneity. Global Change Biology, 12, 822-833. doi:10.1111/j.1365-2486.2006.01137.x | Detail , 2006:
- Asthma epidemiology: Has the crisis passed? Current Opinion in Pulmonary Medicine, 11, 79-84. | Detail , 2005:
- Sensitivity of US air quality to mid-latitude cyclone frequency and implications of 1980–2006 climate change. Atmospheric Chemistry and Physics, 8, 7075-7086. doi:10.5194/acp-8-7075-2008 | Detail , 2008:
- Potential regional climate change and implications to U.S. air quality. Geophysical Research Letters, 32, L16711. doi:10.1029/2005GL022911 | Detail , 2005:
- Chronic exposure to ambient ozone and asthma hospital admissions among children. Environmental Health Perspectives, 116, 1725-1730. doi:10.1289/ehp.11184 | Detail , 2008:
- A systematic review of the physical health impacts from non-occupational exposure to wildfire smoke. Environmental Research, 136, 120-132. doi:10.1016/j.envres.2014.10.015 | Detail , 2015:
- Ch. 9: Human Health. Climate Change Impacts in the United States: The Third National Climate Assessment, , U.S. Global Change Research Program, 220-256. doi:10.7930/J0PN93H5 | Detail , 2014:
- Influence of relative humidity on VOC concentrations in indoor air. Environmental Science and Pollution Research, 22, 5772-5779. doi:10.1007/s11356-014-3678-x | Detail , 2015:
- Temperature, temperature extremes, and mortality: A study of acclimatisation and effect modification in 50 US cities. Occupational and Environmental Medicine, 64, 827-833. doi:10.1136/oem.2007.033175 | Detail , 2007:
- Climate Change Impacts in the United States: The Third National Climate Assessment. U.S. Global Change Research Program, 841 pp. doi:10.7930/J0Z31WJ2 | Detail , 2014:
- California's racial and ethnic minorities more adversely affected by asthma. Policy Brief UCLA Center for Health Policy Research, 1-7. URL | Detail , 2007:
- The effects of race/ethnicity and income on early childhood asthma prevalence and health care use. American Journal of Public Health, 90, 428-430. | Detail , 2000:
- National Surveillance of Asthma: United States, 2001-2010. National Center for Health Statistics. URL | Detail , 2012:
- Public health and economic impact of dampness and mold. Indoor Air, 17, 226-235. doi:10.1111/j.1600-0668.2007.00474.x | Detail , 2007:
- How does climate change contribute to surface ozone change over the United States? Journal of Geophysical Research: Atmospheres, 111, D05301. doi:10.1029/2005JD005873 | Detail , 2006:
- Exploring the consequences of climate change for indoor air quality. Environmental Research Letters, 8, 015022. doi:10.1088/1748-9326/8/1/015022 | Detail , 2013:
- Effects of urbanization on plant flowering phenology: A review. Urban Ecosystems, 9, 243-257. doi:10.1007/s11252-006-9354-2 | Detail , 2006:
- Asthma symptoms in relation to measured building dampness in upper concrete floor construction, and 2-ethyl-1-hexanol in indoor air. International Journal of Tuberculosis and Lung Disease, 4, 1016-1025. URL | Detail , 2000:
- Does nudging squelch the extremes in regional climate modeling? Journal of Climate, 25, 7046-7066. doi:10.1175/JCLI-D-12-00048.1 | Detail , 2012:
- Human activity patterns: A review of the literature for estimating time spent indoors, outdoors, and in transit. Proceedings of the Research Planning Conference on Human Activity Patterns, EPA National Exposure Research Laboratory, 3-1 to 3-38. | Detail , 1989:
- Sources of carbonaceous aerosols over the United States and implications for natural visibility. Journal of Geophysical Research, 108, 4355. doi:10.1029/2002JD003190 | Detail , 2003:
- Legionellosis on the rise: A review of guidelines for prevention in the United States. Journal of Public Health Management and Practice, 21, E17-E26. doi:10.1097/phh.0000000000000123 | Detail , 2015:
- Effect of temperature and humidity on formaldehyde emissions in temporary housing units. Journal of the Air & Waste Management Association, 61, 689-695. doi:10.3155/1047-3289.61.6.689 | Detail , 2011:
- Ambient air pollution and cardiovascular emergency department visits in potentially sensitive groups. American Journal of Epidemiology, 165, 625-633. doi:10.1093/aje/kwk051 | Detail , 2007:
- Impacts of future climate and emission changes on U.S. air quality. Atmospheric Environment, 89, 533-547. doi:10.1016/j.atmosenv.2014.01.001 | Detail , 2014:
- Modeled infiltration rate distributions for U.S. housing. Indoor Air, 20, 473-485. doi:10.1111/j.1600-0668.2010.00669.x | Detail , 2010:
- Projections of future summertime ozone over the U.S. Journal of Geophysical Research: Atmospheres, 119, 5559-5582. doi:10.1002/2013JD020932 | Detail , 2014:
- Epidemiology and clinical management of Legionnaires' disease. The Lancet Infectious Diseases, 14, 1011-1021. doi:10.1016/s1473-3099(14)70713-3 | Detail , 2014:
- Variation in estimated ozone-related health impacts of climate change due to modeling choices and assumptions. Environmental Health Perspectives, 120, 1559-1564. doi:10.1289/ehp.1104271 | Detail , 2012:
- Cardio-respiratory outcomes associated with exposure to wildfire smoke are modified by measures of community health. Environmental Health, 11, Article 71. doi:10.1186/1476-069X-11-71 | Detail , 2012:
- Aeroallergens, allergic disease, and climate change: Impacts and adaptation. EcoHealth, 6, 458-470. doi:10.1007/s10393-009-0261-x | Detail , 2009:
- Does temperature modify short-term effects of ozone on total mortality in 60 large eastern US communities? An assessment using the NMMAPS data. Environment international, 34, 451-458. doi:10.1016/j.envint.2007.10.001 | Detail , 2008:
- Temperature enhanced effects of ozone on cardiovascular mortality in 95 large US communities, 1987-2000: Assessment using the NMMAPS data. Archives of Environmental & Occupational Health, 64, 177-184. doi:10.1080/19338240903240749 | Detail , 2009:
- Ozone modifies associations between temperature and cardiovascular mortality: Analysis of the NMMAPS data. Occupational and Environmental Medicine, 65, 255-260. doi:10.1136/oem.2007.033878 | Detail , 2008:
- Modifiers of short-term effects of ozone on mortality in eastern Massachusetts — A case-crossover analysis at individual level. Environmental Health, 9, Article 3. doi:10.1186/1476-069X-9-3 | Detail , 2010:
- Factors driving hantavirus emergence in Europe. Current Opinion in Virology, 3, 92-99. doi:10.1016/j.coviro.2013.01.002 | Detail , 2013:
- RCP 8.5—A scenario of comparatively high greenhouse gas emissions. Climatic Change, 109, 33-57. doi:10.1007/s10584-011-0149-y | Detail , 2011:
- Projecting policy-relevant metrics for high summertime ozone pollution events over the eastern United States due to climate and emission changes during the 21st century. Journal of Geophysical Research: Atmospheres, 120, 784-800. doi:10.1002/2014JD022303 | Detail , 2015:
- Indoor particulate matter of outdoor origin: Importance of size-dependent removal mechanisms. Environmental Science & Technology, 36, 200-207. doi:10.1021/es010723y | Detail , 2002:
- Phenology in central Europe: Differences and trends of spring phenophases in urban and rural areas. International Journal of Biometeorology, 44, 60-66. doi:10.1007/s004840000062 | Detail , 2000:
- Interaction of the onset of spring and elevated atmospheric CO2 on ragweed (Ambrosia artemisiifolia L.) pollen production. Environmental Health Perspectives, 114, 865-869. doi:10.1289/ehp.8549 | Detail , 2006:
- Particulate matter–induced health effects: Who is susceptible? Environmental Health Perspectives, 119, 446-454. doi:10.1289/ehp.1002255 | Detail , 2011:
- Impact of the 2002 Canadian forest fires on particulate matter air quality in Baltimore City. Environmental Science & Technology, 39, 24-32. doi:10.1021/es035311z | Detail , 2005:
- The impact of climate change and aeroallergens on children's health. Allergy and Asthma Proceedings, 30, 229-237. doi:10.2500/aap.2009.30.3229 | Detail , 2009:
- Model projections of an imminent transition to a more arid climate in southwestern North America. Science, 316, 1181-1184. doi:10.1126/science.1139601 | Detail , 2007:
- Induction of asthma and the environment: What we know and need to know. Environmental Health Perspectives, 114, 615-619. doi:10.1289/ehp.8376 | Detail , 2006:
- Global health and economic impacts of future ozone pollution. Environmental Research Letters, 4, 044014. doi:10.1088/1748-9326/4/4/044014 | Detail , 2009:
- Healthier tribal housing: Combining the best of old and new. Environmental Health Perspectives, 120, A460-A469. doi:10.1289/ehp.120-a460 | Detail , 2012:
- Climate change and allergic disease. Journal of Allergy and Clinical Immunology, 122, 443-453. doi:10.1016/j.jaci.2008.06.032 | Detail , 2008:
- Modeling of regional climate change effects on ground-level ozone and childhood asthma. American Journal of Preventive Medicine, 41, 251-257. doi:10.1016/j.amepre.2011.04.017 | Detail , 2011:
- Climate change, aeroallergens, and pediatric allergic disease. Mount Sinai Journal of Medicine, 78, 78-84. doi:10.1002/msj.20232 | Detail , 2011:
- Increasing Amb a 1 content in common ragweed (Ambrosia artemisiifolia) pollen as a function of rising atmospheric CO2 concentration. Functional Plant Biology, 32, 667-670. doi:10.1071/fp05039 | Detail , 2005:
- Impacts of climate change from 2000 to 2050 on wildfire activity and carbonaceous aerosol concentrations in the western United States. Journal of Geophysical Research: Atmospheres, 114, D20301. doi:10.1029/2008JD010966 | Detail , 2009:
- The climate-wildfire-air quality system: Interactions and feedbacks across spatial and temporal scales. Wiley Interdisciplinary Reviews: Climate Change, 5, 719-733. doi:10.1002/wcc.303 | Detail , 2014:
- Penetration of ambient submicron particles into single-family residences and associations with building characteristics. Indoor Air, 22, 501-513. doi:10.1111/j.1600-0668.2012.00779.x | Detail , 2012:
- Potential impact of climate change on air pollution-related human health effects. Environmental Science & Technology, 43, 4979-4988. doi:10.1021/es803650w | Detail , 2009:
- Impact of 2000–2050 climate change on fine particulate matter (PM2.5) air quality inferred from a multi-model analysis of meteorological modes. Atmospheric Chemistry and Physics, 12, 11329-11337. doi:10.5194/acp-12-11329-2012 | Detail , 2012:
- An overview of CMIP5 and the experiment design. Bulletin of the American Meteorological Society, 93, 485-498. doi:10.1175/BAMS-D-11-00094.1 | Detail , 2012:
- Sensitivity of air quality to potential future climate change and emissions in the United States and major cities. Atmospheric Environment, 94, 552-563. doi:10.1016/j.atmosenv.2014.05.079 | Detail , 2014:
- How emissions, climate, and land use change will impact mid-century air quality over the United States: A focus on effects at National Parks. Atmospheric Chemistry and Physics, 15, 2805-2823. doi:10.5194/acp-15-2805-2015 | Detail , 2015:
- The representative concentration pathways: An overview. Climatic Change, 109, 5-31. doi:10.1007/s10584-011-0148-z | Detail , 2011:
- Carbon monoxide poisoning and flooding: Changes in risk before, during and after flooding require appropriate public health interventions. PLOS Currents: Disasters, July 3, Edition 1. doi:10.1371/currents.dis.2b2eb9e15f9b982784938803584487f1 | Detail , 2014:
- Indoor particles: A review. Journal of the Air & Waste Management Association, 46, 98-126. doi:10.1080/10473289.1996.10467451 | Detail , 1996:
- Ch. 2: Our Changing Climate. Climate Change Impacts in the United States: The Third National Climate Assessment, , U.S. Global Change Research Program, 19-67. doi:10.7930/J0KW5CXT | Detail , 2014:
- The ecology of pneumocystis: Perspectives, personal recollections, and future research opportunities. The Journal of Eukaryotic Microbiology, 60, 634-645. doi:10.1111/jeu.12072 | Detail , 2013:
- Epidemiology of Hantavirus infections in humans: A comprehensive, global overview. Critical Reviews in Microbiology, 40, 261-272. doi:10.3109/1040841x.2013.783555 | Detail , 2014:
- A preliminary synthesis of modeled climate change impacts on U.S. regional ozone concentrations. Bulletin of the American Meteorological Society, 90, 1843-1863. doi:10.1175/2009BAMS2568.1 | Detail , 2009:
- Ozone's impact on public health: Contributions from indoor exposures to ozone and products of ozone-initiated chemistry. Environmental Health Perspectives, 114, 1489-1496. | Detail , 2006:
- Warming and earlier spring increase western U.S. forest wildfire activity. Science, 313, 940-943. doi:10.1126/science.1128834 | Detail , 2006:
- Continued warming could transform Greater Yellowstone fire regimes by mid-21st century. Proceedings of the National Academy of Sciences of the United States of America, 108, 13165-13170. doi:10.1073/pnas.1110199108 | Detail , 2011:
- Opportunistic Pathogens Mycobacterium Avium Complex (MAC) and Legionella spp. Colonise Model Shower. Pathogens, 4, 590-598. doi:10.3390/pathogens4030590 | Detail , 2015:
- WHO Handbook on Indoor Radon: A Public Health Perspective. , World Health Organization, 108 pp. URL | Detail 2009:
- WHO Guidelines for Indoor Air Quality: Selected Pollutants. 484 pp., World Health Organization, Geneva. URL | Detail 2010:
- Effects of 2000–2050 global change on ozone air quality in the United States. Journal of Geophysical Research: Atmospheres, 113, D06302. doi:10.1029/2007JD008917 | Detail , 2008:
- Sources contributing to background surface ozone in the US Intermountain West. Atmospheric Chemistry and Physics, 14, 5295-5309. doi:10.5194/acp-14-5295-2014 | Detail , 2014:
- Development of a regional-scale pollen emission and transport modeling framework for investigating the impact of climate change on allergic airway disease. Biogeosciences, 10, 3977-4023. doi:10.5194/bgd-10-3977-2013 | Detail , 2013:
- Analysis of short-term influences of ambient aeroallergens on pediatric asthma hospital visits. Science of The Total Environment, 370, 330-336. doi:10.1016/j.scitotenv.2006.06.019 | Detail , 2006:
- Impacts of the Bermuda high on regional climate and ozone over the United states. Journal of Climate, 26, 1018-1032. doi:10.1175/JCLI-D-12-00168.1 | Detail , 2013:
- Recent warming by latitude associated with increased length of ragweed pollen season in central North America. Proceedings of the National Academy of Sciences of the United States of America, 108, 4248-4251. doi:10.1073/pnas.1014107108 | Detail , 2011: